Parkinson’s Disease: Linking mitochondria to the immune response
Parkinson’s disease is a motor disorder caused by the loss of a specific sub-set of neurons located in the midbrain and the accumulation of a protein called α-synuclein. The underlying mechanisms that lead to the death of the midbrain neurons are still not well understood. However, many individuals with Parkinson’s disease have increased levels of inflammation in the brain and in peripheral organs, such as the gut. This inflammation is now viewed as a potential contributor to Parkinson’s disease, and not just a result of it.
Mutations in the gene coding for the kinase LRRK2 are the most common genetic cause of inherited Parkinson’s disease (Singleton et al., 2013). Single nucleotide polymorphisms in this gene are also associated with increased susceptibility to inflammatory diseases such as leprosy – which, like tuberculosis, is caused by a mycobacterium – and irritable bowel disease (Fava et al., 2016; Witoelar et al., 2017; Villumsen et al., 2019). Mounting evidence suggests that mutations in LRRK2 contribute to immune alterations in both peripheral organs and the brain (Wallings and Tansey, 2019), but the mechanisms through which LRRK2 regulates these immune responses are poorly understood.
Aside from its link to inflammation, LRRK2 has also been implicated in the activity of mitochondria, which are key subcellular organelles linked to Parkinson’s disease (Singh et al., 2019; Park et al., 2018). For example, neural stem cells derived from the skin of individuals carrying a mutation associated with Parkinson’s disease in the gene for LRRK2 exhibit increased mitochondrial DNA damage as well as increased oxidative damage (Howlett et al., 2017; Sanders et al., 2014). Despite this, the contribution of LRRK2 to mitochondrial health in cells of the peripheral immune system has been understudied. Now, in eLife, Robert Watson and colleagues from Texas A&M University – including Chi Weindel and Samantha Bell as joint first authors – report that LRRK2’s ability to influence inflammatory responses in peripheral immune cells is directly linked to its role in maintaining mitochondrial homeostasis (Weindel et al., 2020).
To investigate the role of LRRK2 in peripheral immune responses, Weindel et al. isolated macrophages from the bone marrow of mice lacking the gene that codes for the mouse homolog of LRRK2 (Lrrk2 knockout macrophages). Compared to macrophages with a single copy of this gene (Lrrk2 HETs), the knockout macrophages upregulated genes normally stimulated by interferons (signaling proteins that recruit immune cells to trigger an immune response). Weindel et al. also found that these macrophages were unable to upregulate interferon response genes when they were infected with mycobacteria, likely because these genes were already chronically activated.
Moreover, when mice lacking Lrrk2 were infected with Mycobacterium tuberculosis, which causes tuberculosis, their lungs exhibited exacerbated local inflammation caused by the infection. However, no differences in the outcome of the infection were observed in these mice relative to the Lrrk2 HETs. This contradicts previous reports suggesting that mice lacking Lrrk2 can stop M. tuberculosis replication more effectively than wild-type mice, correlating with increased inflammation in the lungs (Härtlova et al., 2018). One possible reason for this inconsistency is that Weindel et al. used Lrrk2 heterozygous controls as opposed to wild-type controls. Furthermore, different M. tuberculosis strains were used in the two studies, and there were also differences in the number of bacteria that mice were exposed to during infection.
Since LRRK2 is also involved in mitochondrial activity, Weindel et al. next looked at the mitochondria in Lrrk2 knockout macrophages. Mitochondria are dynamic organelles that can divide (fragment) or fuse depending on the state of the cell. When a cell is stressed, mitochondria fuse together to exchange damaged DNA and keep aerobic respiration going. Weindel et al. observed that in macrophages lacking Lrrk2, mitochondria did not fuse, making the macrophages more susceptible to stress, and leading to mitochondrial DNA leaking into the cytosol. This was due, in part, to increased activation of DRP1, a protein that helps mitochondria fragment. Inhibiting DRP1 successfully rescued these abnormal mitochondria in Lrrk2 knockout macrophages. Weindel et al. also reported reduced antioxidant levels, a concomitant accumulation of reactive oxygen species, and increased mitochondrial stress in macrophages lacking Lrrk2. Treating these macrophages with antioxidants alleviated mitochondrial stress (Figure 1).
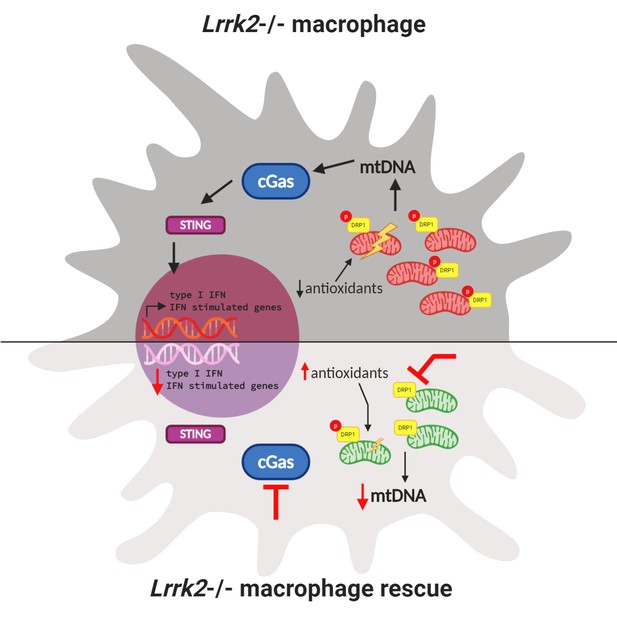
Interactions between mitochondrial homeostasis and immune response in a Lrrk2 knockout macrophage.
(Top) Loss of Lrrk2 in mouse macrophages increases DRP1 phosphorylation. This leads to an increase in the level of mitochondrial DNA (mtDNA; red bean-shaped structures) in the cytosol, which activates the cGAS/STING pathway. This, in turn, triggers the phosphorylation of transcription factors that activate the expression of interferon (IFN) response genes in the nucleus, which leads to a lowered response to interferon from these cells. In this situation, antioxidant levels in the cell are low and mitochondrial stress increases. (Bottom) Three different treatments can partially or totally rescue inflammatory deficits in Lrrk2 knockout macrophages. First, treating these macrophages with antioxidants alleviates mitochondrial stress and rescues the normal response to interferon. Second, inhibiting DRP1 phosphorylation decreases fission, lowering the level of mitochondrial DNA (green bean-shaped structures) in the cytosol. This prevents the activation of the cGAS/STING pathway, and consequently, the abnormal activation of interferon response genes. Third (and confirming this finding), removing the cGas gene also mitigates increased interferon stimulated gene expression.
The increased mitochondrial DNA in the cytosol of Lrrk2 knockout macrophages led Weindel et al. to hypothesize that the inability to regulate mitochondrial homeostasis could be contributing to chronic activation of interferon response genes. The cGAS–STING pathway is a part of the innate immune system that triggers the expression of inflammatory genes when DNA is detected in the cytosol. Weindel et al. showed that loss of the cGas gene decreased basal levels of type I interferon in Lrrk2 knockout macrophages and corrected the response to immune stimuli (Figure 1).
These findings link the role of LRRK2 in innate immune dysregulation with its critical function in maintaining mitochondrial homeostasis for the first time and have wider implications for the field of Parkinson’s disease. Future research will likely investigate how mutations in the gene for LRRK2 affect the relationship between mitochondria and inflammation in immune cells. The most common of these mutations causes a toxic increase in kinase activity, so kinase inhibitors have been considered for their potential therapeutic effects in Parkinson’s disease. However, given that loss of LRRK2 may lead to higher risks of infection and inflammation in peripheral blood cells, therapeutic windows will have to be carefully monitored to avoid unwanted effects on the immune system.
References
-
A missense LRRK2 variant is a risk factor for excessive inflammatory responses in leprosyPLOS Neglected Tropical Diseases 10:e0004412.https://doi.org/10.1371/journal.pntd.0004412
-
Mitochondrial dysfunction in Parkinson's disease: new mechanistic insights and therapeutic perspectivesCurrent Neurology and Neuroscience Reports 18:21.https://doi.org/10.1007/s11910-018-0829-3
-
LRRK2 and mitochondria: recent advances and current viewsBrain Research 1702:96–104.https://doi.org/10.1016/j.brainres.2018.06.010
-
The genetics of Parkinson's disease: progress and therapeutic implicationsMovement Disorders 28:14–23.https://doi.org/10.1002/mds.25249
-
LRRK2 regulation of immune-pathways and inflammatory diseaseBiochemical Society Transactions 47:1581–1595.https://doi.org/10.1042/BST20180463
Article and author information
Author details
Publication history
- Version of Record published: April 15, 2020 (version 1)
Copyright
© 2020, Wallings et al.
This article is distributed under the terms of the Creative Commons Attribution License, which permits unrestricted use and redistribution provided that the original author and source are credited.
Metrics
-
- 2,634
- views
-
- 337
- downloads
-
- 7
- citations
Views, downloads and citations are aggregated across all versions of this paper published by eLife.
Download links
Downloads (link to download the article as PDF)
Open citations (links to open the citations from this article in various online reference manager services)
Cite this article (links to download the citations from this article in formats compatible with various reference manager tools)
Further reading
-
- Immunology and Inflammation
SARS-CoV-2 vaccines have been used worldwide to combat COVID-19 pandemic. To elucidate the factors that determine the longevity of spike (S)-specific antibodies, we traced the characteristics of S-specific T cell clonotypes together with their epitopes and anti-S antibody titers before and after BNT162b2 vaccination over time. T cell receptor (TCR) αβ sequences and mRNA expression of the S-responded T cells were investigated using single-cell TCR- and RNA-sequencing. Highly expanded 199 TCR clonotypes upon stimulation with S peptide pools were reconstituted into a reporter T cell line for the determination of epitopes and restricting HLAs. Among them, we could determine 78 S epitopes, most of which were conserved in variants of concern (VOCs). After the 2nd vaccination, T cell clonotypes highly responsive to recall S stimulation were polarized to follicular helper T (Tfh)-like cells in donors exhibiting sustained anti-S antibody titers (designated as ‘sustainers’), but not in ‘decliners’. Even before vaccination, S-reactive CD4+ T cell clonotypes did exist, most of which cross-reacted with environmental or symbiotic microbes. However, these clonotypes contracted after vaccination. Conversely, S-reactive clonotypes dominated after vaccination were undetectable in pre-vaccinated T cell pool, suggesting that highly responding S-reactive T cells were established by vaccination from rare clonotypes. These results suggest that de novo acquisition of memory Tfh-like cells upon vaccination may contribute to the longevity of anti-S antibody titers.
-
- Chromosomes and Gene Expression
- Immunology and Inflammation
Ikaros is a transcriptional factor required for conventional T cell development, differentiation, and anergy. While the related factors Helios and Eos have defined roles in regulatory T cells (Treg), a role for Ikaros has not been established. To determine the function of Ikaros in the Treg lineage, we generated mice with Treg-specific deletion of the Ikaros gene (Ikzf1). We find that Ikaros cooperates with Foxp3 to establish a major portion of the Treg epigenome and transcriptome. Ikaros-deficient Treg exhibit Th1-like gene expression with abnormal production of IL-2, IFNg, TNFa, and factors involved in Wnt and Notch signaling. While Ikzf1-Treg-cko mice do not develop spontaneous autoimmunity, Ikaros-deficient Treg are unable to control conventional T cell-mediated immune pathology in response to TCR and inflammatory stimuli in models of IBD and organ transplantation. These studies establish Ikaros as a core factor required in Treg for tolerance and the control of inflammatory immune responses.