Plant Reproduction: Long story short
The vast majority of flowering plants are hermaphrodites, with both male and female parts in each of their flowers (Renner, 2014). This strategy is clearly a successful one, coming with several likely advantages including that, by producing both pollen and ovules, each flower has more opportunities to pass genes on to the next generation (Charnov et al., 1976). Although hermaphrodites might also benefit from the possibility of self-fertilization (and many do), most hermaphrodites do all they can to avoid ‘selfing’ because it is often detrimental to a plant’s fitness (Charlesworth and Willis, 2009; Barrett, 2002). Hermaphroditic plants have thus evolved a wonderful array of mechanisms that help them to promote outcrossing.
One of these mechanisms, known as heterostyly, involves male and female parts of the flower (the stamen and the stigma) being in different places in different flowers. The stigma sits on the end of a stalk called the style. Some flowers have long styles and hold their stamens, which produce pollen, deep in the floral tube, whereas others have short styles and hold their stamens much higher in the floral tube (Figure 1A). This means that pollen from a long-styled plant is more likely to pollinate a short-styled flower, and vice versa. Long- and short-styled plants may also differ in the size and colour of their pollen grains and the texture of their stigmas, and outcrossing is only possible between different morphs (Barrett, 2002). Remarkably, despite its complexity, heterostyly has evolved repeatedly in flowering plants and is found in at least 30 families, including the primroses (genus Primula).
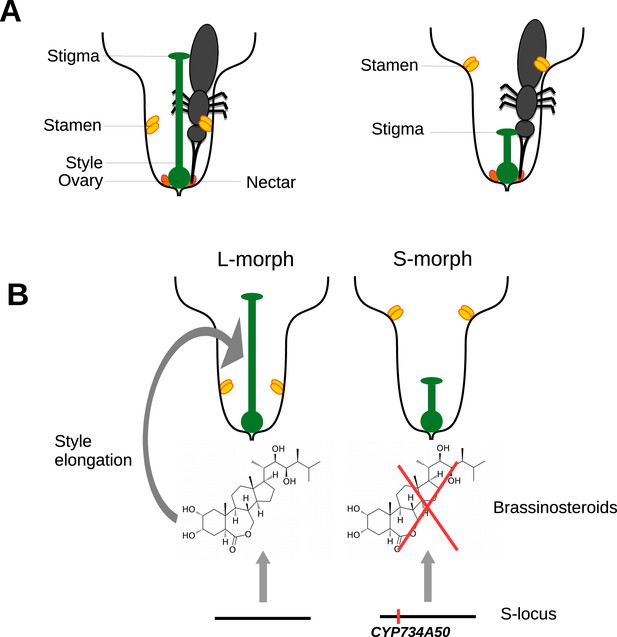
Heterostyly in primroses.
(A) Schematic diagrams of a long-styled (left) and short-styled flower (right) from a distylous species such as Primula vulgaris. When an insect enters a long-styled flower it would pick up pollen mainly on its head. If this insect then visits a short-styled flower, the stigma would pick up the pollen from the insect’s head. Meanwhile, the high stamens would deposit fresh pollen on to the insect’s abdomen for effective transfer to the stigmas of long-styled flowers. (B) Huu et al. identified CYP730A50 as a duplicated gene that alters style length in Primula flowers. Flowers without CYP730A50 (left) produce brassinosteroids, which cause the cells in the style to elongate and produce a long style. On the other hand, flowers with this gene (right) in the S-locus suppress the production of brassinosteroids, which leads to a short style.
Charles Darwin was fascinated by heterostyly (Darwin, 1877) and we now know that it is caused by the expression of several genes that are inherited together as a single genetic unit (locus). However, working out how these genes influence the development of flowers has been something of a holy grail in the field. Now, in eLife, Michael Lenhard at the University of Potsdam and colleagues – including Cuong Nguyen Huu as first author – have identified the gene that is responsible for the different lengths of styles in primroses, and shown how it works (Huu et al., 2016).
Huu et al. found that a gene called CYP734A50 is only present in short-styled flowers in several species of primrose, and estimates of transcript read coverage suggest that only one copy of the gene is present in these flowers. CYP734A50 encodes an enzyme that degrades plant hormones called brassinosteroids, which are known to promote cell elongation (Ohnishi et al., 2006). Huu et al. found that a particular brassinosteroid, castasterone, is present at higher levels in long styles than in short styles, and that treating short styles with another brassinosteroid increased style length (Figure 1B). Moreover, virus-induced silencing of CYP734A50 in young plants with short styles resulted in the production of flowers with longer styles.
It has been thought for a long time, based on theoretical reasoning and comparative analysis, that the first mutation in the evolution of heterostyly was a mutation that reduced style length, and that the expression of this mutated gene should be dominant over the expression of its homologous gene at the style-length locus (Lloyd and Webb, 1992a; 1992b). The results of Huu et al. are consistent with the idea that the expression of the CYP734A50 gene in short-styled individuals is genetically dominant. But how did this dominance come about?
Huu et al. show that CYP734A50 is the result of the duplication of a gene that degrades brassinosteroids more generally. The duplication event occurred early in the evolution of the Primula genus, and the duplicated copy then evolved to be expressed only in styles. The presence or absence of the duplication has been maintained in the genus by a process called negative frequency-dependent selection, whereby the fitness of a trait (e.g. short styles) increases if it becomes rare, thus preventing its loss.
In his short autobiography, Darwin reflected that "I do not think anything in my scientific life has given me so much satisfaction as making out the meaning of the structure of heterostylous flowers". Like so much of his work, the hypothesis that he put forward has stood the test of time and remains the favoured explanation for how and why heterostyly evolved (Barrett, 2002). By identifying the gene that controls style length, the work of Huu et al. is an important step towards understanding the underlying mechanisms involved in heterostyly. A future challenge is to understand the evolutionary history of the other genes involved in heterostyly and to work out what roles they play in flower development.
References
-
The genetics of inbreeding depressionNature Reviews Genetics 10:783–796.https://doi.org/10.1038/nrg2664
-
BookThe evolution of heterostylyIn: Barrett SCH, editors. Evolution and Function of Heterostyly. Berlin: Springer Verlag. pp. 151–178.https://doi.org/10.1007/978-3-642-86656-2_6
-
BookThe selection of heterostylyIn: Barrett SCH, editors. Evolution and Function of Heterostyly. Berlin: Springer Verlag. pp. 179–207.https://doi.org/10.1007/978-3-642-86656-2_7
-
The relative and absolute frequencies of angiosperm sexual systems: dioecy, monoecy, gynodioecy, and an updated online databaseAmerican Journal of Botany 101:1588–1596.https://doi.org/10.3732/ajb.1400196
Article and author information
Author details
Publication history
- Version of Record published: September 6, 2016 (version 1)
- Version of Record updated: September 7, 2016 (version 2)
Copyright
© 2016, Pannell et al.
This article is distributed under the terms of the Creative Commons Attribution License, which permits unrestricted use and redistribution provided that the original author and source are credited.
Metrics
-
- 3,611
- views
-
- 225
- downloads
-
- 0
- citations
Views, downloads and citations are aggregated across all versions of this paper published by eLife.
Download links
Downloads (link to download the article as PDF)
Open citations (links to open the citations from this article in various online reference manager services)
Cite this article (links to download the citations from this article in formats compatible with various reference manager tools)
Further reading
-
- Cancer Biology
- Chromosomes and Gene Expression
The chromatin-associated protein WD Repeat Domain 5 (WDR5) is a promising target for cancer drug discovery, with most efforts blocking an arginine-binding cavity on the protein called the ‘WIN’ site that tethers WDR5 to chromatin. WIN site inhibitors (WINi) are active against multiple cancer cell types in vitro, the most notable of which are those derived from MLL-rearranged (MLLr) leukemias. Peptidomimetic WINi were originally proposed to inhibit MLLr cells via dysregulation of genes connected to hematopoietic stem cell expansion. Our discovery and interrogation of small-molecule WINi, however, revealed that they act in MLLr cell lines to suppress ribosome protein gene (RPG) transcription, induce nucleolar stress, and activate p53. Because there is no precedent for an anticancer strategy that specifically targets RPG expression, we took an integrated multi-omics approach to further interrogate the mechanism of action of WINi in human MLLr cancer cells. We show that WINi induce depletion of the stock of ribosomes, accompanied by a broad yet modest translational choke and changes in alternative mRNA splicing that inactivate the p53 antagonist MDM4. We also show that WINi are synergistic with agents including venetoclax and BET-bromodomain inhibitors. Together, these studies reinforce the concept that WINi are a novel type of ribosome-directed anticancer therapy and provide a resource to support their clinical implementation in MLLr leukemias and other malignancies.
-
- Chromosomes and Gene Expression
- Immunology and Inflammation
Ikaros is a transcriptional factor required for conventional T cell development, differentiation, and anergy. While the related factors Helios and Eos have defined roles in regulatory T cells (Treg), a role for Ikaros has not been established. To determine the function of Ikaros in the Treg lineage, we generated mice with Treg-specific deletion of the Ikaros gene (Ikzf1). We find that Ikaros cooperates with Foxp3 to establish a major portion of the Treg epigenome and transcriptome. Ikaros-deficient Treg exhibit Th1-like gene expression with abnormal production of IL-2, IFNg, TNFa, and factors involved in Wnt and Notch signaling. While Ikzf1-Treg-cko mice do not develop spontaneous autoimmunity, Ikaros-deficient Treg are unable to control conventional T cell-mediated immune pathology in response to TCR and inflammatory stimuli in models of IBD and organ transplantation. These studies establish Ikaros as a core factor required in Treg for tolerance and the control of inflammatory immune responses.