Mutational Scanning: Ion-ing out the genetic variants of Kir2.1
Every time a cell in your body divides, it copies the three billion letter message stored in its DNA. This process is prone to errors and can accidentally introduce mistakes into the genetic code. To counteract this, human cells proofread the copied DNA sequence and correct any inaccuracies (Kunkel, 2004). But typos sometimes fly under the radar, leading to mutations that can alter the overall meaning of the message encoded within the cell’s DNA.
Clinicians can rapidly and easily identify these errors using high-throughput DNA sequencing techniques. However, determining how individual mutations affect the message within DNA is more challenging. This is because some mutations will not change a gene’s activity, while others may substantially impact the function of the protein it encodes, potentially resulting in disease. Traditionally, the best way to study the impact of a mutation was to perform functional studies: researchers made a single mutation, tested it in different types of laboratory experiments, and reported the effect (Jackson et al., 2018). More recently, large-scale analysis techniques that can measure the consequences of all potential mutations in a specific gene have grown in popularity. These approaches (called deep mutational scanning, or DMS for short) allow researchers to make every typo the cell could make in a gene and determine the impact of all such errors in parallel (Fowler et al., 2010).
While DMS has been applied to several genes, these studies have typically only measured a singular output, such as how mutations impact a specific cell behavior or alter the protein encoded within the gene (Jones et al., 2020; Majithia et al., 2016; Starr et al., 2020). Now, in eLife, Willow Coyote-Maestas, David Nedrud, Yungui He and Daniel Schmidt report how they used DMS to characterize how mutations in the gene coding for an ion channel called Kir2.1 impact the function of this protein and its expression simultaneously (Coyote-Maestas et al., 2022).
Kir2.1 belongs to a family of proteins called inward-rectifying potassium channels (Kirs for short) that are studded throughout the cell surface and help stabilize the membrane potential of cells. Mutations in the genes encoding the Kir channels have been linked with various diseases, including diabetes, heart dysfunction, seizures and paralysis (Zangerl-Plessl et al., 2019). However, only a small number of mutations in Kir2.1 have been clinically reported, and how they affect the behavior of the channel is poorly understood.
Coyote-Maestas et al. therefore set out to employ DMS on the Kir2.1 channel, making this the largest disease-associated complex that DMS has ever been applied to. The team (who are based at the University of Minnesota) used this approach to introduce genetic mutations that altered the sequence of amino acids within Kir2.1. Each building block was swapped with every other possible amino acid to create a library of mutants that were then individually inserted into cells grown in the laboratory. To examine how many Kir2.1 channels were expressed at the cell surface, a marker that can be fluorescently labeled was inserted into the genetic sequence of the channel. For changes in functionality, a dye was used that measures membrane potential (Figure 1).
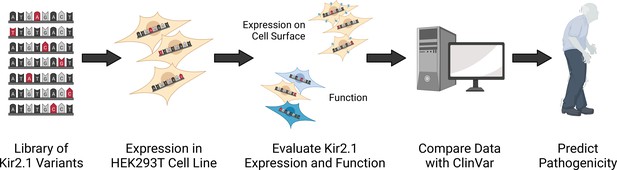
Schematic showing how deep mutational scanning was applied to Kir2.1.
Mutants of the potassium channel Kir2.1 were generated by modifying its DNA sequence so that every amino acid in the protein was swapped for every other possible amino acid. These genetic variants were then integrated and expressed in human cells (called HEK293T) grown in the laboratory. Further experiments were then carried out to determine whether the mutations altered the number of channels expressed on the cell surface, and/or the function of Kir2.1. Mutants were then categorized based on how they impacted the behavior of Kir2.1 and the results were compared to a clinical dataset (called ClinVar) containing mutations found in patients. Genetic variants in the database with no assigned clinical significance were then evaluated and given a pathogenicity score indicating how likely the mutation is to cause disease based on the results from the DMS experiment.
Image credit: Braden Fallon; Figure created using BioRender.
The data revealed that mutations that decreased the expression of Kir2.1 resided in parts of the genome that are responsible for trafficking the channel to the cell surface, or for folding the protein into the right shape. Furthermore, mutations that impacted the formation of the pore in the protein (which allows potassium ions to pass through) were detrimental to the overall expression of Kir2.1. The data also identified segments of Kir2.1 that prevent faulty channels from reaching the cell surface, some of which had already been detected in previous studies (Li et al., 2016; Ma et al., 2011).
Next, Coyote-Maestas et al. investigated changes in the activity of the channel. Interestingly, they found a distinct divide between mutations that impact expression and those that affect functionality. Indeed, many of the mutations that altered how the channel works had no effect on surface expression. This includes a mutation that has been shown to cause Anderson Tawil Syndrome, which is characterized by developmental defects, paralysis, and heart abnormalities (Plaster et al., 2001).
Coyote-Maestas et al. then compared their results to clinical datasets containing mutations in Kir2.1 that had been observed in patients (Figure 1). Each clinically reported mutation was compared to an identical mutation obtained by the group. If the expression or function of the DMS generated mutation was higher or lower than variants which are known to be benign, it was classified as being potentially pathogenic. This allowed Coyote-Maestas et al. to predict whether variants that have not yet been clinically observed are likely to cause disease, and determine how mutations with unclear or uncertain clinical effects may impact the function and expression of Kir2.1.
The comparison also revealed that most clinically reported mutations were associated with the protein’s function rather than its ability to successfully reach and embed itself into the cell surface. As Kir2.1 is needed for many aspects of physiology, mutations that significantly impact its expression are likely to be fatal (Zaritsky et al., 2001), which may be why these types of variants are less common in the general public.
Taken together, these findings suggest that DMS can provide various mutational insights. First, it can be used to predict the clinical significance of variants with previously unknown impacts. Second, it can be used to predict regions in DNA where mutations are more likely to occur and be clinically observed. Finally, this approach can predict how a mutation will impact a protein’s cellular role. However, while this study provides a significant breadth of new information, it is worth noting that only one experiment was performed to characterize the functional consequences of the mutations studied. Additional assays are needed, including in vivo studies, to validate the pathological effect of these genetic variants.
This work demonstrates how DMS can be used to study multiple parameters. Future DMS studies will benefit from mirroring this approach, and considering how mutations impact both expression and function simultaneously. The methodology established by Coyote-Maestas et al. can be broadly applied to other ion channels, and lays the groundworks for advancing DMS studies in many biochemical applications.
References
-
DNA replication fidelityThe Journal of Biological Chemistry 279:16895–16898.https://doi.org/10.1074/jbc.R400006200
-
A common signal patch drives AP-1 protein-dependent Golgi export of inwardly rectifying potassium channelsThe Journal of Biological Chemistry 291:14963–14972.https://doi.org/10.1074/jbc.M116.729822
-
Prospective functional classification of all possible missense variants in PPARGNature Genetics 48:1570–1575.https://doi.org/10.1038/ng.3700
Article and author information
Author details
Publication history
- Version of Record published: July 11, 2022 (version 1)
Copyright
© 2022, Fallon and English
This article is distributed under the terms of the Creative Commons Attribution License, which permits unrestricted use and redistribution provided that the original author and source are credited.
Metrics
-
- 555
- views
-
- 113
- downloads
-
- 0
- citations
Views, downloads and citations are aggregated across all versions of this paper published by eLife.
Download links
Downloads (link to download the article as PDF)
Open citations (links to open the citations from this article in various online reference manager services)
Cite this article (links to download the citations from this article in formats compatible with various reference manager tools)
Further reading
-
- Genetics and Genomics
Uncovering the regulators of cellular aging will unravel the complexity of aging biology and identify potential therapeutic interventions to delay the onset and progress of chronic, aging-related diseases. In this work, we systematically compared genesets involved in regulating the lifespan of Saccharomyces cerevisiae (a powerful model organism to study the cellular aging of humans) and those with expression changes under rapamycin treatment. Among the functionally uncharacterized genes in the overlap set, YBR238C stood out as the only one downregulated by rapamycin and with an increased chronological and replicative lifespan upon deletion. We show that YBR238C and its paralog RMD9 oppositely affect mitochondria and aging. YBR238C deletion increases the cellular lifespan by enhancing mitochondrial function. Its overexpression accelerates cellular aging via mitochondrial dysfunction. We find that the phenotypic effect of YBR238C is largely explained by HAP4- and RMD9-dependent mechanisms. Furthermore, we find that genetic- or chemical-based induction of mitochondrial dysfunction increases TORC1 (Target of Rapamycin Complex 1) activity that, subsequently, accelerates cellular aging. Notably, TORC1 inhibition by rapamycin (or deletion of YBR238C) improves the shortened lifespan under these mitochondrial dysfunction conditions in yeast and human cells. The growth of mutant cells (a proxy of TORC1 activity) with enhanced mitochondrial function is sensitive to rapamycin whereas the growth of defective mitochondrial mutants is largely resistant to rapamycin compared to wild type. Our findings demonstrate a feedback loop between TORC1 and mitochondria (the TORC1–MItochondria–TORC1 (TOMITO) signaling process) that regulates cellular aging processes. Hereby, YBR238C is an effector of TORC1 modulating mitochondrial function.
-
- Genetics and Genomics
- Neuroscience
Adenine phosphoribosyltransferase (APRT) and hypoxanthine-guanine phosphoribosyltransferase (HGPRT) are two structurally related enzymes involved in purine recycling in humans. Inherited mutations that suppress HGPRT activity are associated with Lesch–Nyhan disease (LND), a rare X-linked metabolic and neurological disorder in children, characterized by hyperuricemia, dystonia, and compulsive self-injury. To date, no treatment is available for these neurological defects and no animal model recapitulates all symptoms of LND patients. Here, we studied LND-related mechanisms in the fruit fly. By combining enzymatic assays and phylogenetic analysis, we confirm that no HGPRT activity is expressed in Drosophila melanogaster, making the APRT homolog (Aprt) the only purine-recycling enzyme in this organism. Whereas APRT deficiency does not trigger neurological defects in humans, we observed that Drosophila Aprt mutants show both metabolic and neurobehavioral disturbances, including increased uric acid levels, locomotor impairments, sleep alterations, seizure-like behavior, reduced lifespan, and reduction of adenosine signaling and content. Locomotor defects could be rescued by Aprt re-expression in neurons and reproduced by knocking down Aprt selectively in the protocerebral anterior medial (PAM) dopaminergic neurons, the mushroom bodies, or glia subsets. Ingestion of allopurinol rescued uric acid levels in Aprt-deficient mutants but not neurological defects, as is the case in LND patients, while feeding adenosine or N6-methyladenosine (m6A) during development fully rescued the epileptic behavior. Intriguingly, pan-neuronal expression of an LND-associated mutant form of human HGPRT (I42T), but not the wild-type enzyme, resulted in early locomotor defects and seizure in flies, similar to Aprt deficiency. Overall, our results suggest that Drosophila could be used in different ways to better understand LND and seek a cure for this dramatic disease.