Drug Discovery: Decoding the mechanisms of allostery
G protein-coupled receptors are transmembrane proteins that help to regulate a wide array of biological processes, which makes them important drug targets. However, different receptors often share a high similarity in their sequences, especially at their binding sites, which often results in challenges to develop drugs that target a specific receptor (Vuckovic et al., 2019; Singh and Karnik, 2021).
For example, the five members of a muscarinic acetylcholine receptor subfamily (M1-M5 mAChR) have essential roles in various physiological processes (Wess et al., 2007). In particular, M4 mAChR is of major therapeutic interest due to its involvement in regulating dopaminergic neurons involved in cognition, psychosis and addiction, while others, such as M1 mAChR, can be targeted to treat cognitive decline in Alzheimer’s disease (Wess et al., 2007). However, these receptors share highly similar binding sites, and drugs that target a particular mAChR receptor often inadvertently activate other receptors in the subfamily, thereby causing adverse side effects (Felder et al., 2018).
As an alternative to targeting the primary binding site on the receptor (also known as the orthosteric site) with a drug, it is sometimes possible to regulate a receptor by targeting a remote (or allosteric) site. Since there is much less similarity in the sequences of allosteric sites, this approach makes it possible to design highly selective drugs with reduced side effects.
Now, in eLife, David Thal, Arthur Christopoulus and Celine Valant (all at Monash University), Yinglong Miao (University of Kansas) and colleagues – including Ziva Vuckovic, Vi Pham and Jesse Mobbs (all at Monash) and Jinan Wang (Kansas) as joint first authors, along with colleagues in Japan, the United Kingdom and the United States – report on the molecular mechanisms that govern allostery in human M4 AchR (Vuckovic et al., 2023). The researchers used two ligands that targeted the orthosteric site (acetylcholine and iperoxo), and two positive modulators that targeted the allosteric site (VU154 and LY298). Both modulators have shown antipsychotic efficacy in preclinical rodent models, but these results have failed to translate into human studies (Suratman et al., 2011; Dupuis et al., 2010). Nevertheless, they remain useful tools for investigating allostery in G protein-coupled receptors (Bubser et al., 2014).
Vuckovic et al. used two types of biochemical assays to determine the pharmacological characteristics of the allosteric modulators. This revealed that both LY298 and VU154 display a phenomenon called ‘probe dependence’, meaning that they had a stronger effect when the orthosteric ligand was acetylcholine rather than iperoxo. They also showed that these effects were caused by an increase in the binding affinities of the orthosteric ligands, rather than by any modulation of signaling through the receptor (Figure 1A). Moreover, LY298 was the more potent modulator as it caused a 400-fold increase in binding affinity, compared with a modest 40-fold increase for VU154.
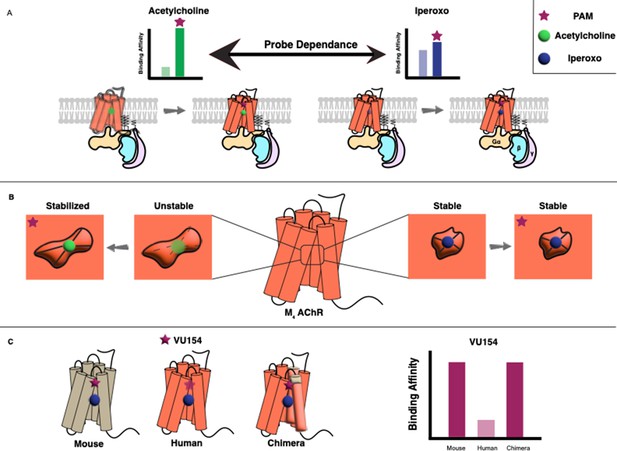
Allosteric regulation of G protein-coupled receptors.
(A) G protein-coupled receptors (GPCRs) are transmembrane proteins (bottom) that can be regulated by orthosteric ligands (such as acetylcholine or iperoxo) and positive allosteric modulators (PAMs). Vuckovic et al. studied a receptor called M4 AChR and found that two PAMs (VU154 or LY298; purple stars) bind more tightly when the orthosteric ligand is acetylcholine (green circle), and less tightly when it is iperoxo (blue circle); this is referred to as “probe dependance”. (B) This probe dependence can be explained by differences in the binding of acetylcholine and iperoxo at the orthosteric site, which is inside a ‘pocket’. This pocket contracts around iperoxo, which results in iperoxo and the allosteric ligand forming a stable complex, but it does not contract around acetylcholine, which results in a more dynamic complex. (C) The ability of VU154 to bind to the receptor varies from species to species and is higher in mice compared to humans. However, introducing mutations to make the genetic sequence in the human receptor more like that of the mouse receptor led to an increase in binding affinity.
To uncover the molecular basis for these results, Vuckovic et al. used cryogenic electron microscopy (cryoEM) to obtain four structures of M4 AChR bound to its cognate G protein and in complex with various ligands. The structural analyses – combined with molecular dynamics simulations – enabled the authors to uncover the underlying dynamics and conformational changes that are otherwise missed through static snapshots of cryoEM structures.
The experiments revealed that the allosteric sites for both VU154 and LY298 were, as expected, located in a region of the receptor called the extracellular vestibule. The orthosteric sites overlapped with those in other members of the mAChR subfamily and were located inside a central ‘pocket’ in the receptor; However, it was noticed that this pocket was contracted around iperoxo but not around acetylcholine. The smaller binding pocket, along with the rotation of a specific tyrosine residue, resulted in more stable interactions for iperoxo within the orthosteric site. On the other hand, the binding of acetylcholine was seen to be more dynamic with fewer stable interactions (Figure 1B).
Surprisingly, even though iperoxo bound to the receptor more tightly than acetylcholine, its ability to promote signaling through the receptor was lower. Vuckovic et al. suggest that since the acetylcholine-bound M4 AChR is more dynamic, it can sample a large range of conformations, including those that couple to and activate G protein. This allows the receptor to efficiently activate the G protein and increase the signaling response.
The structures and molecular dynamics simulations also helped uncover the molecular basis for the probe dependence of the allosteric modulators. It is possible that both had stronger effects on the acetylcholine-bound receptor due to the stabilization of an inherently dynamic structure. Conversely, since the iperoxo-bound receptor was already very stable, the modulatory effects were negligible. This result provides a key future consideration for the development of allosteric drugs that target G protein-coupled receptors.
Using mutational studies, Vuckovic et al. also identified a network of amino acids that were important to the conformational dynamics of the protein, some of which showed maximum variability between structures and modulated the signaling efficacy of both orthosteric and allosteric ligands.
Lastly, the researchers investigated why VU154 is potent in some species but not in others. Based on their initial findings, VU154 was a weaker positive allosteric modulator than LY298 in humans because it poorly stabilized the active receptor conformation. However, its effects in mice were stronger, and were comparable to the effects of LY298 in humans. Using mutational studies, Vuckovic et al. identified three important residues on the human receptor that confer species-selectivity. Mutating these to the equivalent residues in the mouse sequence resulted in improved allostery by VU154 in functional studies and stable binding in simulations.
In conclusion, Vuckovic et al. have described the complex interplay between structure, conformational dynamics and pharmacology that defines allostery at G protein-coupled receptors. Their work provides a detailed framework to guide future drug discovery efforts focused on the muscarinic receptor subfamily.
References
-
Current trends in GPCR allosteryThe Journal of Membrane Biology 254:293–300.https://doi.org/10.1007/s00232-020-00167-6
-
DataStructure of the M5 muscarinic acetylcholine receptor (M5-T4L)Worldwide Protein Data Bank.https://doi.org/10.2210/pdb6ol9/pdb
-
Muscarinic acetylcholine receptors: mutant mice provide new insights for drug developmentNature Reviews. Drug Discovery 6:721–733.https://doi.org/10.1038/nrd2379
Article and author information
Author details
Publication history
- Version of Record published: June 2, 2023 (version 1)
Copyright
© 2023, Khan and Gati
This article is distributed under the terms of the Creative Commons Attribution License, which permits unrestricted use and redistribution provided that the original author and source are credited.
Metrics
-
- 799
- views
-
- 92
- downloads
-
- 1
- citations
Views, downloads and citations are aggregated across all versions of this paper published by eLife.
Download links
Downloads (link to download the article as PDF)
Open citations (links to open the citations from this article in various online reference manager services)
Cite this article (links to download the citations from this article in formats compatible with various reference manager tools)
Further reading
-
- Biochemistry and Chemical Biology
- Cell Biology
Hibernation is a period of metabolic suppression utilized by many small and large mammal species to survive during winter periods. As the underlying cellular and molecular mechanisms remain incompletely understood, our study aimed to determine whether skeletal muscle myosin and its metabolic efficiency undergo alterations during hibernation to optimize energy utilization. We isolated muscle fibers from small hibernators, Ictidomys tridecemlineatus and Eliomys quercinus and larger hibernators, Ursus arctos and Ursus americanus. We then conducted loaded Mant-ATP chase experiments alongside X-ray diffraction to measure resting myosin dynamics and its ATP demand. In parallel, we performed multiple proteomics analyses. Our results showed a preservation of myosin structure in U. arctos and U. americanus during hibernation, whilst in I. tridecemlineatus and E. quercinus, changes in myosin metabolic states during torpor unexpectedly led to higher levels in energy expenditure of type II, fast-twitch muscle fibers at ambient lab temperatures (20 °C). Upon repeating loaded Mant-ATP chase experiments at 8 °C (near the body temperature of torpid animals), we found that myosin ATP consumption in type II muscle fibers was reduced by 77–107% during torpor compared to active periods. Additionally, we observed Myh2 hyper-phosphorylation during torpor in I. tridecemilineatus, which was predicted to stabilize the myosin molecule. This may act as a potential molecular mechanism mitigating myosin-associated increases in skeletal muscle energy expenditure during periods of torpor in response to cold exposure. Altogether, we demonstrate that resting myosin is altered in hibernating mammals, contributing to significant changes to the ATP consumption of skeletal muscle. Additionally, we observe that it is further altered in response to cold exposure and highlight myosin as a potentially contributor to skeletal muscle non-shivering thermogenesis.
-
- Biochemistry and Chemical Biology
- Neuroscience
In most mammals, conspecific chemosensory communication relies on semiochemical release within complex bodily secretions and subsequent stimulus detection by the vomeronasal organ (VNO). Urine, a rich source of ethologically relevant chemosignals, conveys detailed information about sex, social hierarchy, health, and reproductive state, which becomes accessible to a conspecific via vomeronasal sampling. So far, however, numerous aspects of social chemosignaling along the vomeronasal pathway remain unclear. Moreover, since virtually all research on vomeronasal physiology is based on secretions derived from inbred laboratory mice, it remains uncertain whether such stimuli provide a true representation of potentially more relevant cues found in the wild. Here, we combine a robust low-noise VNO activity assay with comparative molecular profiling of sex- and strain-specific mouse urine samples from two inbred laboratory strains as well as from wild mice. With comprehensive molecular portraits of these secretions, VNO activity analysis now enables us to (i) assess whether and, if so, how much sex/strain-selective ‘raw’ chemical information in urine is accessible via vomeronasal sampling; (ii) identify which chemicals exhibit sufficient discriminatory power to signal an animal’s sex, strain, or both; (iii) determine the extent to which wild mouse secretions are unique; and (iv) analyze whether vomeronasal response profiles differ between strains. We report both sex- and, in particular, strain-selective VNO representations of chemical information. Within the urinary ‘secretome’, both volatile compounds and proteins exhibit sufficient discriminative power to provide sex- and strain-specific molecular fingerprints. While total protein amount is substantially enriched in male urine, females secrete a larger variety at overall comparatively low concentrations. Surprisingly, the molecular spectrum of wild mouse urine does not dramatically exceed that of inbred strains. Finally, vomeronasal response profiles differ between C57BL/6 and BALB/c animals, with particularly disparate representations of female semiochemicals.