Synapses: Changing the size of dendritic spines
Neurons connect to one another at sites called synapses, which are essential for creating and storing memories. Synapses are also dynamic and can undergo structural changes, such as increasing in size, that modify their strength. Structures called dendritic spines – which protrude from the dendrites that extend from the cell body of a neuron – have an important role in synapses as they contain an actin cytoskeleton that allows them to change shape and size.
An enzyme called CaMKII (which is short for Ca2+/calmodulin-dependent protein kinase II) also has a central role in the functional and structural changes that take place in dendritic spines during memory formation. During synapse strengthening, an influx of calcium ions through a receptor known as NMDAR, triggers the activation of CaMKII: the calcium ions form a complex with a messenger protein called calmodulin, and this complex binds to the regulatory segment of CaMKII. This influences the dynamics of the actin cytoskeleton and hence determines the size of the synapses. However, the details of this process – especially the link between CaMKII and the actin cytoskeleton at the molecular level – are not fully understood.
One candidate for linking CaMKII to the actin cytoskeleton is alpha-actinin-2, a structural protein that crosslinks actin filaments (Walikonis et al., 2001). This protein can anchor and organize other proteins in the dendritic spine, such as CaMKII (Robison et al., 2005), the receptor NMDAR (Wyszynski et al., 1997), and certain channel proteins that allow calcium ions to enter cells (Hall et al., 2013). Now, in eLife, Matthew Gold from University College London and colleagues – including Ashton Curtis and Jian Zhu as joint first authors – report new insights into the molecular interactions between CaMKII, alpha-actinin-2, and a subunit of NMDAR called GluN2B (Curtis et al., 2023; Figure 1).
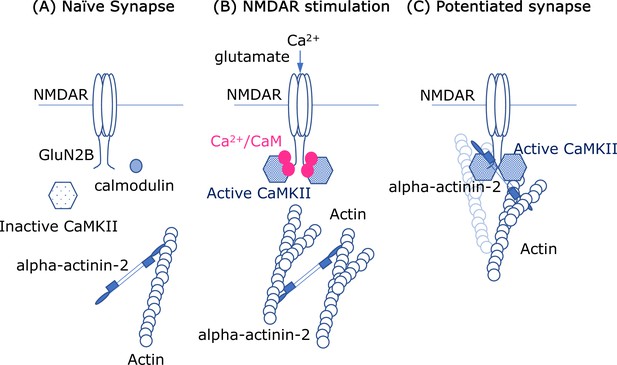
Molecular interactions and synapses.
(A) In the naïve synapse, the only protein interactions are between actin filaments (blue) and alpha-actinin-2, a structural protein that crosslinks actin filaments. In particular, the enzyme CaMKII (hexagon shape) is inactive, and a messenger protein called calmodulin (CaM) does not interact with anything else. (B) When the NMDAR receptor in the cell membrane is activated by the neurotransmitter glutamate, calcium ions (Ca2+; solid pink circles) enter the cell. The ions form a complex with CaM, and this complex activates the enzyme CaMKII, which subsequently interacts with the GluN2B subunits in the intracellular region of the NMDAR receptor. Crosslinking of the actin filaments also causes the dendritic spine to increase in size. (C) In the enlarged synapse, the interaction between GluN2B, CaMKII and alpha-actinin-2 continues, even in the absence of Ca2+/CaM, and the enzymatic activity of CaMKII is maintained by binding to GluN2B. The complex formed by GluN2B, CaMKII and alpha-actinin-2 also associates with actin filaments to maintain the synaptic structure.
Using a proximity labeling assay method – which enables molecular interactions to be detected in situ – the researchers showed that CaMKII interacts with alpha-actinin-2 when the NMDAR receptor is stimulated, leading to an enlargement of the dendritic spine. The interaction takes place at a domain in alpha-actinin-2 called EF hands 1–4, which is known to bind calcium ions. Furthermore, when this domain was overexpressed, stimulation of the NMDAR receptor did not result in dendritic spine enlargement, suggesting that interaction via the EF1-4 domain has a critical role in structural changes to the dendritic spine.
Affinity and structural experiments showed that alpha-actinin-2 binds to the CaMKII enzyme at the same regulatory domain segment as calmodulin (which also contains EF hands) does. However, Curtis et al. found that the EF hands of alpha-actinin-2 clashed with one of the domains in the enzyme. Consistent with this, the inactive form of the enzyme cannot bind to alpha-actinin-2 via its regulatory domain segment. However, the interaction between the CaMKII enzyme and alpha-actinin-2 in the dendritic spine increased four hours after stimulation of the NMDAR receptor: this led Curtis et al. to hypothesize that, following stimulation, the configuration of the enzyme changes when it interacts with a subunit of the NMDAR receptor called GluN2B, and these changes enable alpha-actinin-2 to fully access the enzyme.
A pull-down assay – which detects interactions between proteins – revealed that GluN2B enhances the interaction between the CaMKII enzyme and alpha-actinin-2, independently of the concentration of calcium ions (Ca2+). This indicates that GluN2B enhances the binding of CaMKII with alpha-actinin-2 even in the presence of Ca2+/calmodulin, although the EF hands of alpha-actinin-2 have a lower affinity for CaMKII than the EF hands of Ca2+/calmodulin. This provides important insights into a reorganization process for the molecular architecture of synapses.
Taken together, the findings suggest that the tripartite interactions of CaMKII, GluN2B, and alpha-actinin-2 crosslink a membrane channel to the cytoskeleton beneath the synapse, and there are plenty of empty binding sites that other proteins can bind to. Moreover, the fact that certain interactions would generate autonomous enzymatic activity in CaMKII (Bayer et al., 2001; Saneyoshi et al., 2019) suggest a possible role in biochemical signalling for synaptic memory.
Recent evidence indicates that the CaMKII and GluN2B in synapses can undergo liquid–liquid phase separation, a process that involves biomolecules separating into distinct components within a cell (Hosokawa et al., 2021; Yasuda et al., 2022). Actin bundling can also be modulated by liquid–liquid phase separation in synapses (Chen et al., 2023), so it would be interesting to explore if this process has a role in changing and/or maintaining the structure of dendritic spines.
References
-
Multivalent interactions of calcium/calmodulin-dependent protein kinase II with the postsynaptic density proteins NR2B, densin-180, and alpha-actinin-2The Journal of Biological Chemistry 280:35329–35336.https://doi.org/10.1074/jbc.M502191200
-
CaMKII: a central molecular organizer of synaptic plasticity, learning and memoryNature Reviews Neuroscience 23:666–682.https://doi.org/10.1038/s41583-022-00624-2
Article and author information
Author details
Publication history
- Version of Record published: September 7, 2023 (version 1)
Copyright
© 2023, Saneyoshi
This article is distributed under the terms of the Creative Commons Attribution License, which permits unrestricted use and redistribution provided that the original author and source are credited.
Metrics
-
- 808
- views
-
- 73
- downloads
-
- 0
- citations
Views, downloads and citations are aggregated across all versions of this paper published by eLife.
Download links
Downloads (link to download the article as PDF)
Open citations (links to open the citations from this article in various online reference manager services)
Cite this article (links to download the citations from this article in formats compatible with various reference manager tools)
Further reading
-
- Neuroscience
The fragile X syndrome (FXS) represents the most prevalent form of inherited intellectual disability and is the first monogenic cause of autism spectrum disorder. FXS results from the absence of the RNA-binding protein FMRP (fragile X messenger ribonucleoprotein). Neuronal migration is an essential step of brain development allowing displacement of neurons from their germinal niches to their final integration site. The precise role of FMRP in neuronal migration remains largely unexplored. Using live imaging of postnatal rostral migratory stream (RMS) neurons in Fmr1-null mice, we observed that the absence of FMRP leads to delayed neuronal migration and altered trajectory, associated with defects of centrosomal movement. RNA-interference-induced knockdown of Fmr1 shows that these migratory defects are cell-autonomous. Notably, the primary Fmrp mRNA target implicated in these migratory defects is microtubule-associated protein 1B (MAP1B). Knocking down MAP1B expression effectively rescued most of the observed migratory defects. Finally, we elucidate the molecular mechanisms at play by demonstrating that the absence of FMRP induces defects in the cage of microtubules surrounding the nucleus of migrating neurons, which is rescued by MAP1B knockdown. Our findings reveal a novel neurodevelopmental role for FMRP in collaboration with MAP1B, jointly orchestrating neuronal migration by influencing the microtubular cytoskeleton.
-
- Biochemistry and Chemical Biology
- Neuroscience
In most mammals, conspecific chemosensory communication relies on semiochemical release within complex bodily secretions and subsequent stimulus detection by the vomeronasal organ (VNO). Urine, a rich source of ethologically relevant chemosignals, conveys detailed information about sex, social hierarchy, health, and reproductive state, which becomes accessible to a conspecific via vomeronasal sampling. So far, however, numerous aspects of social chemosignaling along the vomeronasal pathway remain unclear. Moreover, since virtually all research on vomeronasal physiology is based on secretions derived from inbred laboratory mice, it remains uncertain whether such stimuli provide a true representation of potentially more relevant cues found in the wild. Here, we combine a robust low-noise VNO activity assay with comparative molecular profiling of sex- and strain-specific mouse urine samples from two inbred laboratory strains as well as from wild mice. With comprehensive molecular portraits of these secretions, VNO activity analysis now enables us to (i) assess whether and, if so, how much sex/strain-selective ‘raw’ chemical information in urine is accessible via vomeronasal sampling; (ii) identify which chemicals exhibit sufficient discriminatory power to signal an animal’s sex, strain, or both; (iii) determine the extent to which wild mouse secretions are unique; and (iv) analyze whether vomeronasal response profiles differ between strains. We report both sex- and, in particular, strain-selective VNO representations of chemical information. Within the urinary ‘secretome’, both volatile compounds and proteins exhibit sufficient discriminative power to provide sex- and strain-specific molecular fingerprints. While total protein amount is substantially enriched in male urine, females secrete a larger variety at overall comparatively low concentrations. Surprisingly, the molecular spectrum of wild mouse urine does not dramatically exceed that of inbred strains. Finally, vomeronasal response profiles differ between C57BL/6 and BALB/c animals, with particularly disparate representations of female semiochemicals.